What is the Working Principle of High-Voltage Capacitors?
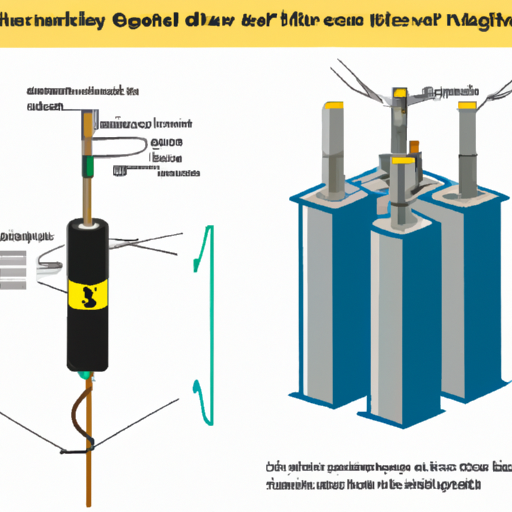
I. Introduction
High-voltage capacitors are essential components in various electrical systems, playing a crucial role in energy storage, power transmission, and industrial applications. These capacitors are designed to operate at voltages significantly higher than standard capacitors, making them vital for applications where high energy storage and rapid discharge are required. This article will explore the working principles of high-voltage capacitors, their applications, design considerations, challenges, and future trends in technology.
II. Basic Principles of Capacitors
A. Definition and Function of Capacitors
A capacitor is an electronic component that stores electrical energy in an electric field. It consists of two conductive plates separated by an insulating material known as a dielectric. When a voltage is applied across the plates, an electric field is created, allowing the capacitor to store energy.
B. Components of a Capacitor
1. **Plates**: The conductive plates are typically made of metals such as aluminum or tantalum. The surface area of these plates directly affects the capacitance of the capacitor.
2. **Dielectric Material**: The dielectric is the insulating material between the plates, which can be made from various substances, including ceramics, plastics, or electrolytes. The choice of dielectric material significantly influences the capacitor's performance and voltage rating.
C. Capacitance and Its Measurement
1. **Formula for Capacitance**: The capacitance (C) of a capacitor is defined by the formula:
\[
C = \frac{Q}{V}
\]
where \(Q\) is the charge stored in coulombs, and \(V\) is the voltage across the plates in volts.
2. **Factors Affecting Capacitance**: Capacitance is influenced by the surface area of the plates, the distance between them, and the properties of the dielectric material. Increasing the plate area or using a dielectric with a higher permittivity will increase capacitance.
III. High-Voltage Capacitors: An Overview
A. Definition and Characteristics of High-Voltage Capacitors
High-voltage capacitors are designed to operate at voltages typically exceeding 1,000 volts. They are built to withstand the stresses associated with high voltage, including dielectric breakdown and thermal effects. These capacitors are characterized by their ability to store large amounts of energy and discharge it rapidly when needed.
B. Applications of High-Voltage Capacitors
1. **Power Transmission and Distribution**: High-voltage capacitors are used in power systems to improve voltage stability, reduce losses, and enhance the efficiency of power transmission.
2. **Energy Storage Systems**: They play a critical role in energy storage systems, such as those used in renewable energy applications, where they store energy generated from sources like solar and wind.
3. **Industrial Applications**: High-voltage capacitors are utilized in various industrial applications, including motor drives, power factor correction, and inverters.
C. Types of High-Voltage Capacitors
1. **Ceramic Capacitors**: Known for their stability and reliability, ceramic capacitors are often used in high-voltage applications due to their excellent dielectric properties.
2. **Film Capacitors**: These capacitors use thin plastic films as dielectrics and are favored for their high voltage ratings and low losses.
3. **Electrolytic Capacitors**: While typically used for lower voltage applications, specialized high-voltage electrolytic capacitors are available for specific uses, particularly in energy storage.
IV. Working Principle of High-Voltage Capacitors
A. Charging Process
1. **Voltage Application**: When a voltage is applied across the plates of a high-voltage capacitor, electrons accumulate on one plate, creating a negative charge, while the other plate loses electrons, resulting in a positive charge.
2. **Electric Field Formation**: The separation of charges creates an electric field between the plates, which is proportional to the applied voltage. This electric field is what allows the capacitor to store energy.
B. Energy Storage Mechanism
1. **Role of Dielectric Material**: The dielectric material between the plates enhances the capacitor's ability to store energy. It polarizes in response to the electric field, which increases the overall capacitance.
2. **Polarization Effects**: The polarization of the dielectric material reduces the electric field strength within the capacitor, allowing it to store more charge at a given voltage.
C. Discharging Process
1. **Release of Stored Energy**: When the capacitor is connected to a load, the stored energy is released as current flows from one plate to the other, powering the connected circuit.
2. **Current Flow and Circuit Behavior**: The discharge process can be rapid, depending on the load, and is characterized by an exponential decay of current over time, governed by the circuit's resistance and capacitance.
V. Design Considerations for High-Voltage Capacitors
A. Dielectric Materials Used
1. **Properties of Dielectric Materials**: High-voltage capacitors require dielectrics with high breakdown voltages, low losses, and good thermal stability. Common materials include polypropylene, polyester, and ceramic.
2. **Selection Criteria for High-Voltage Applications**: The choice of dielectric material is critical, as it must withstand high electric fields without breaking down or degrading over time.
B. Construction Techniques
1. **Insulation and Safety Measures**: High-voltage capacitors must be designed with robust insulation to prevent electrical breakdown and ensure safety during operation.
2. **Size and Form Factor Considerations**: The physical size of high-voltage capacitors can vary significantly based on their application, with larger capacitors typically used for energy storage and smaller ones for circuit applications.
C. Thermal Management
1. **Heat Generation During Operation**: High-voltage capacitors can generate heat during operation due to dielectric losses. Effective thermal management is essential to maintain performance and longevity.
2. **Cooling Methods**: Various cooling methods, including air cooling, liquid cooling, and heat sinks, are employed to dissipate heat and maintain optimal operating temperatures.
VI. Challenges and Limitations
A. Voltage Breakdown and Dielectric Failure
One of the primary challenges in high-voltage capacitors is the risk of voltage breakdown, where the dielectric material fails, leading to short circuits and potential damage to the capacitor and surrounding components.
B. Aging and Degradation of Capacitors
Over time, high-voltage capacitors can experience aging and degradation due to environmental factors, electrical stress, and thermal cycling, which can affect their performance and reliability.
C. Environmental Factors Affecting Performance
High-voltage capacitors are susceptible to environmental conditions such as humidity, temperature fluctuations, and exposure to contaminants, which can impact their lifespan and functionality.
VII. Future Trends and Innovations
A. Advances in Materials Science
Research in materials science is leading to the development of new dielectric materials with improved properties, enabling higher voltage ratings and better performance in high-voltage capacitors.
B. Emerging Technologies in Capacitor Design
Innovations in capacitor design, such as the use of nanotechnology and advanced manufacturing techniques, are paving the way for more efficient and compact high-voltage capacitors.
C. Potential Applications in Renewable Energy Systems
As the demand for renewable energy sources grows, high-voltage capacitors will play a crucial role in energy storage systems, helping to stabilize power grids and improve the efficiency of renewable energy integration.
VIII. Conclusion
In summary, high-voltage capacitors are vital components in modern electrical systems, enabling efficient energy storage and power transmission. Understanding their working principles, design considerations, and challenges is essential for engineers and technicians working in the field. As technology advances, high-voltage capacitors will continue to evolve, offering new solutions for energy storage and management in an increasingly electrified world.
IX. References
A comprehensive list of academic journals, industry publications, and textbooks on electrical engineering and capacitor technology would be included here to support the information presented in the article.
---
This blog post provides a detailed exploration of high-voltage capacitors, their working principles, applications, and future trends, making it a valuable resource for anyone interested in electrical engineering and capacitor technology.
What is the Working Principle of High-Voltage Capacitors?
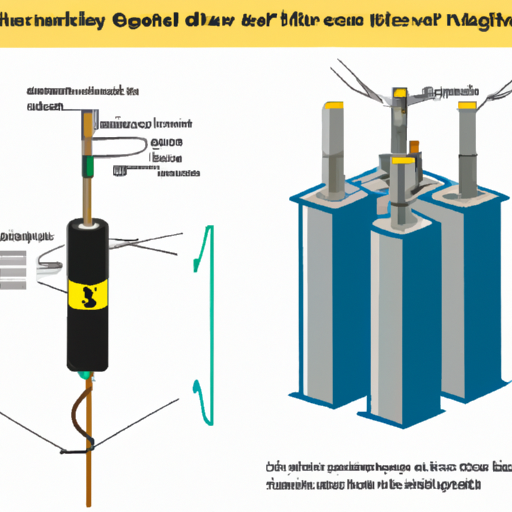
I. Introduction
High-voltage capacitors are essential components in various electrical systems, playing a crucial role in energy storage, power transmission, and industrial applications. These capacitors are designed to operate at voltages significantly higher than standard capacitors, making them vital for applications where high energy storage and rapid discharge are required. This article will explore the working principles of high-voltage capacitors, their applications, design considerations, challenges, and future trends in technology.
II. Basic Principles of Capacitors
A. Definition and Function of Capacitors
A capacitor is an electronic component that stores electrical energy in an electric field. It consists of two conductive plates separated by an insulating material known as a dielectric. When a voltage is applied across the plates, an electric field is created, allowing the capacitor to store energy.
B. Components of a Capacitor
1. **Plates**: The conductive plates are typically made of metals such as aluminum or tantalum. The surface area of these plates directly affects the capacitance of the capacitor.
2. **Dielectric Material**: The dielectric is the insulating material between the plates, which can be made from various substances, including ceramics, plastics, or electrolytes. The choice of dielectric material significantly influences the capacitor's performance and voltage rating.
C. Capacitance and Its Measurement
1. **Formula for Capacitance**: The capacitance (C) of a capacitor is defined by the formula:
\[
C = \frac{Q}{V}
\]
where \(Q\) is the charge stored in coulombs, and \(V\) is the voltage across the plates in volts.
2. **Factors Affecting Capacitance**: Capacitance is influenced by the surface area of the plates, the distance between them, and the properties of the dielectric material. Increasing the plate area or using a dielectric with a higher permittivity will increase capacitance.
III. High-Voltage Capacitors: An Overview
A. Definition and Characteristics of High-Voltage Capacitors
High-voltage capacitors are designed to operate at voltages typically exceeding 1,000 volts. They are built to withstand the stresses associated with high voltage, including dielectric breakdown and thermal effects. These capacitors are characterized by their ability to store large amounts of energy and discharge it rapidly when needed.
B. Applications of High-Voltage Capacitors
1. **Power Transmission and Distribution**: High-voltage capacitors are used in power systems to improve voltage stability, reduce losses, and enhance the efficiency of power transmission.
2. **Energy Storage Systems**: They play a critical role in energy storage systems, such as those used in renewable energy applications, where they store energy generated from sources like solar and wind.
3. **Industrial Applications**: High-voltage capacitors are utilized in various industrial applications, including motor drives, power factor correction, and inverters.
C. Types of High-Voltage Capacitors
1. **Ceramic Capacitors**: Known for their stability and reliability, ceramic capacitors are often used in high-voltage applications due to their excellent dielectric properties.
2. **Film Capacitors**: These capacitors use thin plastic films as dielectrics and are favored for their high voltage ratings and low losses.
3. **Electrolytic Capacitors**: While typically used for lower voltage applications, specialized high-voltage electrolytic capacitors are available for specific uses, particularly in energy storage.
IV. Working Principle of High-Voltage Capacitors
A. Charging Process
1. **Voltage Application**: When a voltage is applied across the plates of a high-voltage capacitor, electrons accumulate on one plate, creating a negative charge, while the other plate loses electrons, resulting in a positive charge.
2. **Electric Field Formation**: The separation of charges creates an electric field between the plates, which is proportional to the applied voltage. This electric field is what allows the capacitor to store energy.
B. Energy Storage Mechanism
1. **Role of Dielectric Material**: The dielectric material between the plates enhances the capacitor's ability to store energy. It polarizes in response to the electric field, which increases the overall capacitance.
2. **Polarization Effects**: The polarization of the dielectric material reduces the electric field strength within the capacitor, allowing it to store more charge at a given voltage.
C. Discharging Process
1. **Release of Stored Energy**: When the capacitor is connected to a load, the stored energy is released as current flows from one plate to the other, powering the connected circuit.
2. **Current Flow and Circuit Behavior**: The discharge process can be rapid, depending on the load, and is characterized by an exponential decay of current over time, governed by the circuit's resistance and capacitance.
V. Design Considerations for High-Voltage Capacitors
A. Dielectric Materials Used
1. **Properties of Dielectric Materials**: High-voltage capacitors require dielectrics with high breakdown voltages, low losses, and good thermal stability. Common materials include polypropylene, polyester, and ceramic.
2. **Selection Criteria for High-Voltage Applications**: The choice of dielectric material is critical, as it must withstand high electric fields without breaking down or degrading over time.
B. Construction Techniques
1. **Insulation and Safety Measures**: High-voltage capacitors must be designed with robust insulation to prevent electrical breakdown and ensure safety during operation.
2. **Size and Form Factor Considerations**: The physical size of high-voltage capacitors can vary significantly based on their application, with larger capacitors typically used for energy storage and smaller ones for circuit applications.
C. Thermal Management
1. **Heat Generation During Operation**: High-voltage capacitors can generate heat during operation due to dielectric losses. Effective thermal management is essential to maintain performance and longevity.
2. **Cooling Methods**: Various cooling methods, including air cooling, liquid cooling, and heat sinks, are employed to dissipate heat and maintain optimal operating temperatures.
VI. Challenges and Limitations
A. Voltage Breakdown and Dielectric Failure
One of the primary challenges in high-voltage capacitors is the risk of voltage breakdown, where the dielectric material fails, leading to short circuits and potential damage to the capacitor and surrounding components.
B. Aging and Degradation of Capacitors
Over time, high-voltage capacitors can experience aging and degradation due to environmental factors, electrical stress, and thermal cycling, which can affect their performance and reliability.
C. Environmental Factors Affecting Performance
High-voltage capacitors are susceptible to environmental conditions such as humidity, temperature fluctuations, and exposure to contaminants, which can impact their lifespan and functionality.
VII. Future Trends and Innovations
A. Advances in Materials Science
Research in materials science is leading to the development of new dielectric materials with improved properties, enabling higher voltage ratings and better performance in high-voltage capacitors.
B. Emerging Technologies in Capacitor Design
Innovations in capacitor design, such as the use of nanotechnology and advanced manufacturing techniques, are paving the way for more efficient and compact high-voltage capacitors.
C. Potential Applications in Renewable Energy Systems
As the demand for renewable energy sources grows, high-voltage capacitors will play a crucial role in energy storage systems, helping to stabilize power grids and improve the efficiency of renewable energy integration.
VIII. Conclusion
In summary, high-voltage capacitors are vital components in modern electrical systems, enabling efficient energy storage and power transmission. Understanding their working principles, design considerations, and challenges is essential for engineers and technicians working in the field. As technology advances, high-voltage capacitors will continue to evolve, offering new solutions for energy storage and management in an increasingly electrified world.
IX. References
A comprehensive list of academic journals, industry publications, and textbooks on electrical engineering and capacitor technology would be included here to support the information presented in the article.
---
This blog post provides a detailed exploration of high-voltage capacitors, their working principles, applications, and future trends, making it a valuable resource for anyone interested in electrical engineering and capacitor technology.